- Joined
- Jul 21, 2007
- Messages
- 34
- Points
- 0
Blackbody Radiation
The Sun and our position relative to it is quite possibly the single most important asset the human race has, and ever will, have. It provides a source of gravity that lockes our planet in the 'goldilocks zone' and provides energy, that in the goldilocks zone, is perfect to allow the spontaneous creation and evolution of biological organisums. The most prominat feature though, is light. Visible electromagnetic radiation that wakes us up in the morning ans sends us to bed at night. One of the many gifts 'granted' to us more complex organisums is the ability to see the world around us by examining the structure of this electromagnetic radiation. Why though, given that the electromagnetic spectrum is incredibly large, are we confined to what we call the visible range? Why not Ultraviolet? Why not Infrared or X-Ray?
The answer is in how the Sun emmits its light, which is quite different to how lasers emmit theirs but virtually identical to the common lightbulb or candle. Understanding the different processes that can be used to create electromagnetic radiation, including light, can be quite interesting. I do not have the time to give you full mathematical descriptions of each process, that is an almost impossible task, but I do intend to at least show you where the math comes from, what it means, and how it relates to our world.
To start, consider that large ball of fire we do call the Sun. A enormous ball of hydrogen, helium and other light elements constantly fusing together in complex reaction to release huge amounts of energy. Surely the mechanisum produceing light is equally complex. Or not. It turns out that any charged particle that accellerates (changes speed or direction) will emit radiation, the amount of accelleration determining the frequency of the radiation emitted. Atoms are charged particles and vibrate when given energy - heat. If an atom has any temperature above absolute zero, then they will vibrate and emit radiation. The higher the temperature, the higher the energy, the faster the vibration and the higher the frequency of emmitted radiation. This is called blackbody raidation.
Historicly, we have known of the suns spectrum for quite some time, but not until relatively recently were we able to find a mathmatical model for it that explain what was physically happening. In 1859, Gustav Kirchhoff discovered that the energy emitted from a blackbody system depended only on frequency and temperature, but he could not find a function to describe it. Instead, he put out an open invitation for other physicists to help solve the problem. And solve they did. Wilhelm Wien produced an approximation for the longer wavelengths, and Lord Rayleigh and Sir James Jeans produced another approximation for the shorter wavelengths. It was not until Max Planck in 1901 proposed that emitted radiation was quantised that the full law for blackbody radiation was discovered. Unlike the approximations that covered either side of the spectrum seen from a radiating body, Planck's Law is a full solution and matches exactly what is seen in experimentation.
The human body is at a temperature of 309K (Kelvin). This is a temperature above absolute zero, and so we must emmit radiation. Putting 309K into Planck's Law and we get far infrared light at a peak of 9000nm, but with components from 3000nm upto 25000nm and more. This is what thermal imaging cameras detect, the quality depending as usual on how much you want to spend on detectors. Police do not need to see your acne, a white human figure is good enough. For scientists though, more detail is requires, at a cost. Below is the spectrum from a human at 309K, and an image showing the difference between far infrared and visible, taken from the blackbody wikipedia link above.
The hotter objects get, the more the spectrum moves to the left. Not to have failed on his previous attempt at solving the problem, Wein provided a usefull law called Wein's Displacement Law that shows how the peak wavelength changed with temperature. This is a much easier calculation than calculating Planck's law and finding the peak, and provides a reasonably accurate value of the peak emmitted wavelength.
Crank the temperature up to that of say, an incandescent lightbulb which lies at around 2500K, and you move into the near infrared range with only part in the visible (400nm-700nm). This is why they are inefficient. Most energy is released in near infrared radiaiton that we feel as heat, but do not see. Halogen lamps burn hotter at about 3100K with the addition of halogen gases to prevent damage from the higher temperature. This pushes the spectrum further into the Visible, making them more efficient as light sources. Both can be seen below.
The Sun takes it one further, with a surface temperature of 5800K. This gives a peak wavelength just about on 500nm, allowing for some to fall in the blue around 400nm and plenty in the red at 600nm+ to give it that yellowish look. This blackbody radiation from the sun is without a doubt the largest source of energy to our planet. It is not then hard to understand that nature has evolved to make the most of it. If you are going to take energy from the sun to aid growth, such as in plants, you might want to set your cells up to absorb the wavelengths that are most abundant. Similarly, if you are going to use electromagnetic radiation to view the world around you, it is best to centre your system on the wavelengths that are again more prominant. The spectral response of the eye was taken from here.
Unfortunately, raising and lowering the temperature of materials can not be used to produce all frequencies of radiation in usefull amounts. As you get hotter, you dramatically increase the chance of damaging the material and keeping it in confinement. As you go lower, you decrease the amount of energy available for radiation. While it may be at the right frequency, there just is not enough of it to do anything. In both cases, it is also hard to keep equipment at that temperature, making it expensive and hard to maintain. The other issue is that blackbody radiation is broadband radiation. That is, it emmits radiation over a large range of frequencies. This is usefull for applications such as lighting (but not good enough for say, museums that want natural light without having to have a light burning at 5800K). It is not usefull however, for most scientific purpouses that require specific wavelengths. Other issues arrise from the size of the devices relative to the power they output, and longativity.
Part 2 will delve a little deeper into the spectrum of the Sun, and how it relates to the lasers we love and the advantages in light generation that can be achieved.
Edit: If the pictures don't fit in your browser, let me know and I will resize. My screens, note the s, are kinda big and have no respect for the little guys.
The Sun and our position relative to it is quite possibly the single most important asset the human race has, and ever will, have. It provides a source of gravity that lockes our planet in the 'goldilocks zone' and provides energy, that in the goldilocks zone, is perfect to allow the spontaneous creation and evolution of biological organisums. The most prominat feature though, is light. Visible electromagnetic radiation that wakes us up in the morning ans sends us to bed at night. One of the many gifts 'granted' to us more complex organisums is the ability to see the world around us by examining the structure of this electromagnetic radiation. Why though, given that the electromagnetic spectrum is incredibly large, are we confined to what we call the visible range? Why not Ultraviolet? Why not Infrared or X-Ray?
The answer is in how the Sun emmits its light, which is quite different to how lasers emmit theirs but virtually identical to the common lightbulb or candle. Understanding the different processes that can be used to create electromagnetic radiation, including light, can be quite interesting. I do not have the time to give you full mathematical descriptions of each process, that is an almost impossible task, but I do intend to at least show you where the math comes from, what it means, and how it relates to our world.
To start, consider that large ball of fire we do call the Sun. A enormous ball of hydrogen, helium and other light elements constantly fusing together in complex reaction to release huge amounts of energy. Surely the mechanisum produceing light is equally complex. Or not. It turns out that any charged particle that accellerates (changes speed or direction) will emit radiation, the amount of accelleration determining the frequency of the radiation emitted. Atoms are charged particles and vibrate when given energy - heat. If an atom has any temperature above absolute zero, then they will vibrate and emit radiation. The higher the temperature, the higher the energy, the faster the vibration and the higher the frequency of emmitted radiation. This is called blackbody raidation.
Historicly, we have known of the suns spectrum for quite some time, but not until relatively recently were we able to find a mathmatical model for it that explain what was physically happening. In 1859, Gustav Kirchhoff discovered that the energy emitted from a blackbody system depended only on frequency and temperature, but he could not find a function to describe it. Instead, he put out an open invitation for other physicists to help solve the problem. And solve they did. Wilhelm Wien produced an approximation for the longer wavelengths, and Lord Rayleigh and Sir James Jeans produced another approximation for the shorter wavelengths. It was not until Max Planck in 1901 proposed that emitted radiation was quantised that the full law for blackbody radiation was discovered. Unlike the approximations that covered either side of the spectrum seen from a radiating body, Planck's Law is a full solution and matches exactly what is seen in experimentation.
The human body is at a temperature of 309K (Kelvin). This is a temperature above absolute zero, and so we must emmit radiation. Putting 309K into Planck's Law and we get far infrared light at a peak of 9000nm, but with components from 3000nm upto 25000nm and more. This is what thermal imaging cameras detect, the quality depending as usual on how much you want to spend on detectors. Police do not need to see your acne, a white human figure is good enough. For scientists though, more detail is requires, at a cost. Below is the spectrum from a human at 309K, and an image showing the difference between far infrared and visible, taken from the blackbody wikipedia link above.
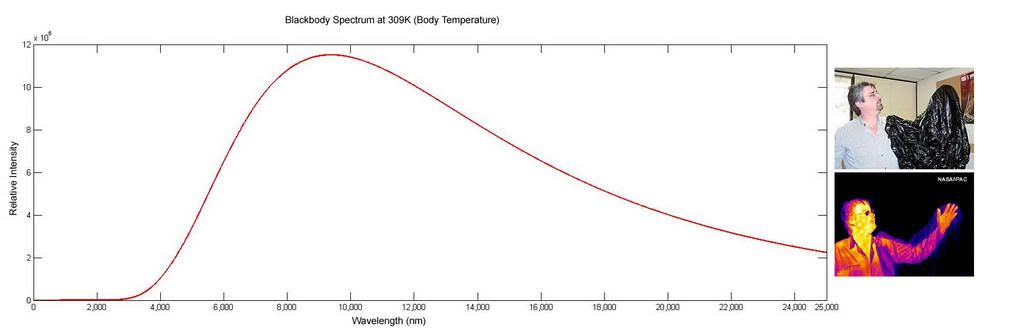
The hotter objects get, the more the spectrum moves to the left. Not to have failed on his previous attempt at solving the problem, Wein provided a usefull law called Wein's Displacement Law that shows how the peak wavelength changed with temperature. This is a much easier calculation than calculating Planck's law and finding the peak, and provides a reasonably accurate value of the peak emmitted wavelength.
Crank the temperature up to that of say, an incandescent lightbulb which lies at around 2500K, and you move into the near infrared range with only part in the visible (400nm-700nm). This is why they are inefficient. Most energy is released in near infrared radiaiton that we feel as heat, but do not see. Halogen lamps burn hotter at about 3100K with the addition of halogen gases to prevent damage from the higher temperature. This pushes the spectrum further into the Visible, making them more efficient as light sources. Both can be seen below.
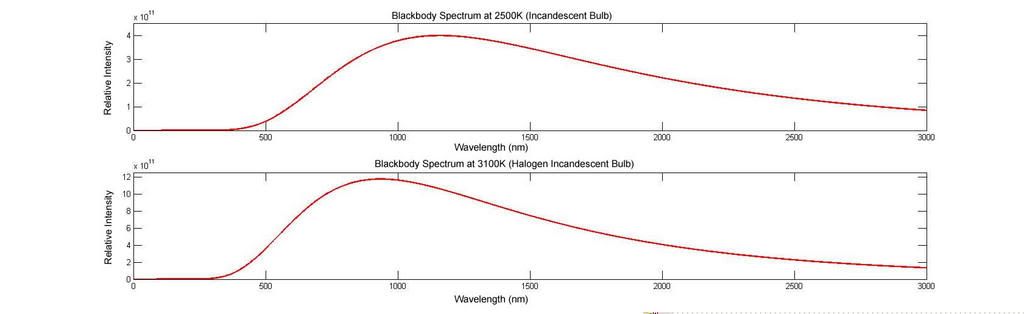
The Sun takes it one further, with a surface temperature of 5800K. This gives a peak wavelength just about on 500nm, allowing for some to fall in the blue around 400nm and plenty in the red at 600nm+ to give it that yellowish look. This blackbody radiation from the sun is without a doubt the largest source of energy to our planet. It is not then hard to understand that nature has evolved to make the most of it. If you are going to take energy from the sun to aid growth, such as in plants, you might want to set your cells up to absorb the wavelengths that are most abundant. Similarly, if you are going to use electromagnetic radiation to view the world around you, it is best to centre your system on the wavelengths that are again more prominant. The spectral response of the eye was taken from here.
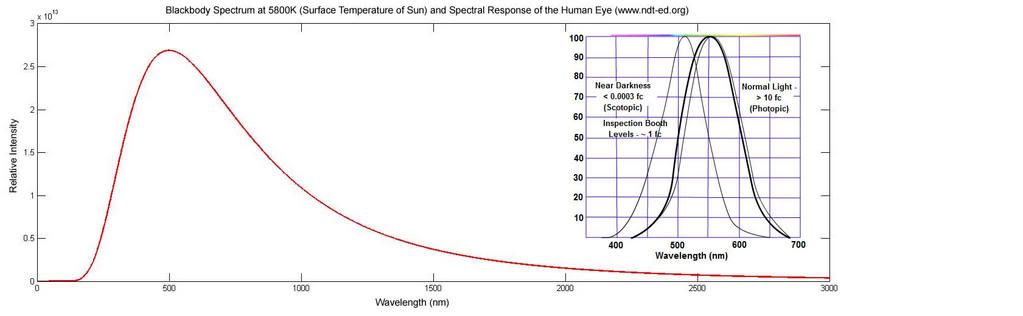
Unfortunately, raising and lowering the temperature of materials can not be used to produce all frequencies of radiation in usefull amounts. As you get hotter, you dramatically increase the chance of damaging the material and keeping it in confinement. As you go lower, you decrease the amount of energy available for radiation. While it may be at the right frequency, there just is not enough of it to do anything. In both cases, it is also hard to keep equipment at that temperature, making it expensive and hard to maintain. The other issue is that blackbody radiation is broadband radiation. That is, it emmits radiation over a large range of frequencies. This is usefull for applications such as lighting (but not good enough for say, museums that want natural light without having to have a light burning at 5800K). It is not usefull however, for most scientific purpouses that require specific wavelengths. Other issues arrise from the size of the devices relative to the power they output, and longativity.
Part 2 will delve a little deeper into the spectrum of the Sun, and how it relates to the lasers we love and the advantages in light generation that can be achieved.
Edit: If the pictures don't fit in your browser, let me know and I will resize. My screens, note the s, are kinda big and have no respect for the little guys.